Raumweise Heizlastberechnung: Der Weg zur optimalen Wärmepumpe
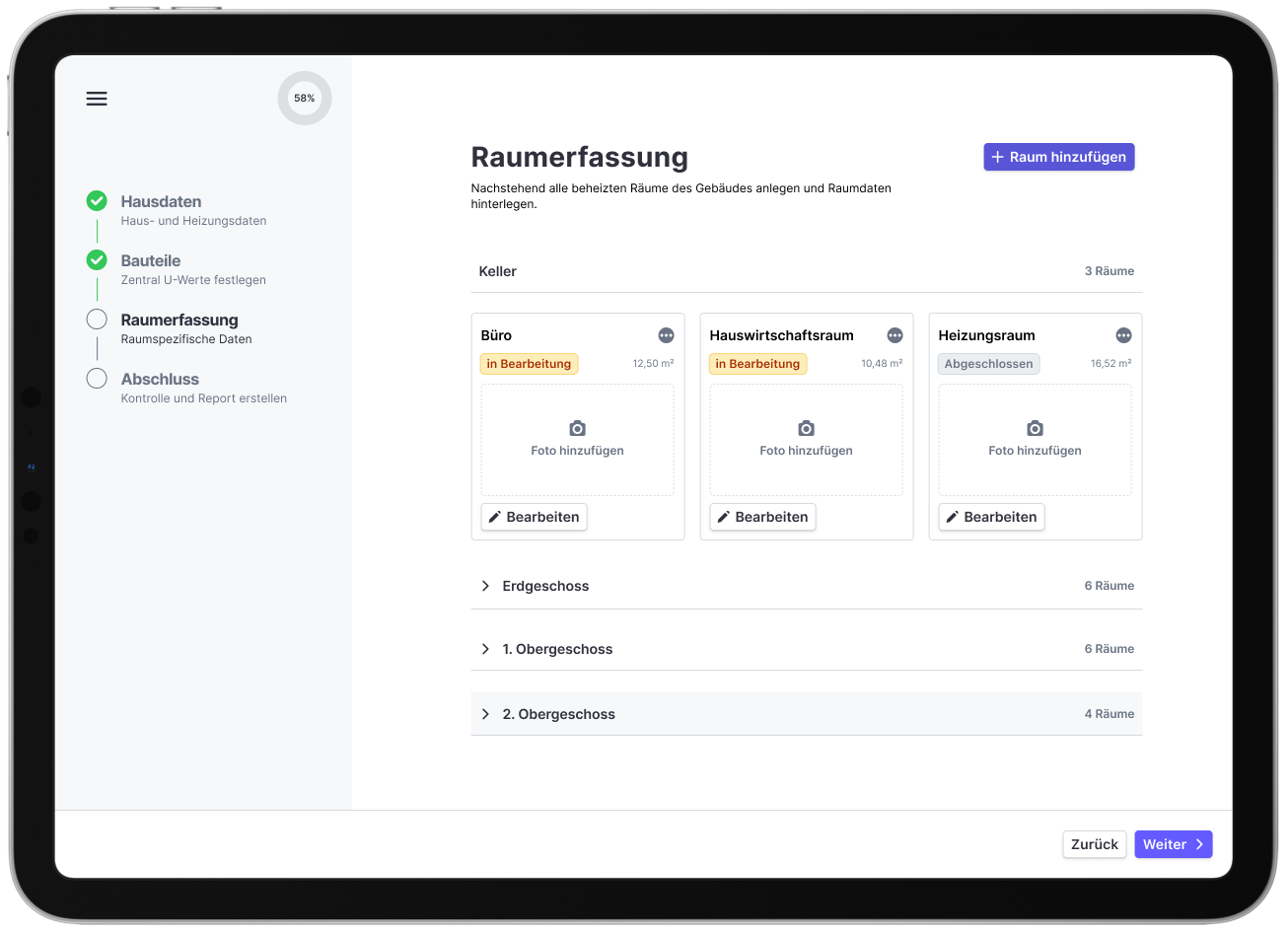
Heute, wo jeder Quadratmeter und jede Energieeinheit zählt, ganz im Sinne von Energieeffizienz, Umweltschutz und maximierten Wohnkomfort, kommen Handwerksbetriebe, Energieberater und Hausbesitzer um die raumweise Heizlastberechnung nicht herum. Doch was genau verbirgt sich hinter diesem Begriff? Und warum spielt er eine so entscheidende Rolle bei der Wahl einer optimalen Wärmepumpe?
Die raumweise Heizlastberechnung dient dazu, den genauen Wärmebedarf für jeden Raum in einem Gebäude zu bestimmen. Sie ist nicht nur ein technisches Muss, sondern stellt auch sicher, dass jede Ecke unseres Zuhauses genau die richtige Menge an Wärme erhält. In diesem Beitrag werden wir diesen Prozess zerlegen, seine Rolle bei der Wahl der richtigen Wärmepumpe klären und erklären, warum diese scheinbar kleine Berechnung einen großen Einfluss auf unsere Umwelt und unseren Geldbeutel haben kann. Schauen wir uns also die Wärme in unseren Räumen einmal genauer an und warum es mehr braucht als nur einen Thermostat, um ein energieeffizientes Zuhause zu schaffen.
Grundlagen der Heizlastberechnung: Warum ist sie unerlässlich?
Unterschied zwischen Heizlast und raumweise Heizlastberechnung
Die Heizlast oder auch Heizleistung bezeichnet jene Energiemenge, die die Heizungsanlage benötigt, um ein Gebäude auch an den kältesten Tagen des Jahres auf eine Zimmertemperatur von ca. 20-22° C zu erwärmen und zu halten. Sie ist quasi die "Leistung", die unsere Heizsysteme erfüllen müssen. Dabei ist zu beachten, dass es nicht nur darum geht, Wärmeverluste auszugleichen, sondern auch, sich an wechselnde klimatische Bedingungen und unterschiedliche Nutzungsmuster der Räume anzupassen. Diese Wärmezufuhr wird in Watt (W) oder Kilowatt (kW) angegeben und berücksichtigt regionale Unterschiede, wobei sie je nach genauem Ort für Außentemperaturen (Klimakarte) von ca. -10°C und kälter kalkuliert wird. Durch gezielte Wärmeschutzmaßnahmen lässt sich die Heizlast effektiv reduzieren.
Die raumweise Heizlastberechnung geht noch einen Schritt weiter. Statt eine allgemeine Berechnung für das gesamte Gebäude vorzunehmen, betrachtet sie jeden Raum einzeln. Das bedeutet, dass die spezifischen Anforderungen und Bedingungen jedes Raumes – wie Größe, Isolierung, Anzahl und Art der Fenster und viele andere Faktoren – in die Berechnung einfließen. Das Ergebnis ist eine maßgeschneiderte Heizlösung für jeden Raum, die sowohl Komfort als auch Effizienz gewährleistet. Damit können alle Heizflächen so ausgelegt werden, dass jeder Raum nach seinem tatsächlichen Wärmebedarf beheizt werden kann.
Leider wird die Heizlast in vielen Fällen nicht korrekt bestimmt. In Deutschland haben Studien der Deutschen Bundesstiftung Umwelt gezeigt, dass Heizkessel oft fast doppelt bis dreimal so groß sind, wie sie eigentlich sein müssten (Link). Dies führt nicht nur zu höheren Anschaffungskosten, sondern auch zu ineffizienterem Betrieb und somit zu mehr Kosten im Alltag. Hier einige Probleme, die dadurch entstehen:
- Die Pumpen im System sind überdimensioniert und verbrauchen unnötig viel Strom.
- Moderne, effiziente Heizkessel arbeiten nicht bei ihrer besten Leistung (erhöhter Verschleiss).
- Das Nutzerverhalten, die Reglereinstellung und hydraulische Einbindung des Wärmeerzeugers sind zusätzlich zu beachten. Mehr hierzu in unserem Beitrag zum hydraulischen Abgleich nach dem Verfahren B.
Bei der Modernisierung von Heizkesseln ist es daher unerlässlich, die aktuelle Heizlast zur Bestimmung der Leistung des Wärmeerzeugers genau zu ermitteln. Dies gilt insbesondere für ältere Gebäude, bei denen oft keine Dokumentation über die Wärmedurchgangskoeffizienten (U-Werte, früher als k-Werte bezeichnet) vorliegt. Historisch betrachtet sind viele ältere Heizkessel tendenziell überdimensioniert, und mit der Zeit wurden die Gebäude energetisch optimiert – durch Maßnahmen wie Außendämmung oder den Einbau neuer Fenster. Daher ist es riskant, die Heizleistung des vorherigen Kessels blindlings und ohne weiteren Berechnungen zu übernehmen.
Die Normen DIN EN 12831:2017 und DIN/TS 12831-1:2020-04
Um sicherzustellen, dass die Heizlastberechnung auch korrekt durchgeführt wird, folgt sie einem standardisierten Verfahren, das in der DIN EN 12831 festgelegt ist.
Die DIN EN 12831:2017 ist eine europäische Norm, die das Verfahren zur Bestimmung der Norm-Heizlast von Einzelräumen, Gebäudeteilen und kompletten Gebäuden. Dabei wird die Norm-Heizlast als jene Wärmeleistung definiert, die erforderlich ist, um die vorgegebene Norm-Innentemperatur unter festgelegten Norm-Außenbedingungen zu gewährleisten.
Die DIN/TS 12831-1:2020-04 ist eine technische Spezifikation, die spezifisch für die raumweise Heizlastberechnung entwickelt wurde. Sie basiert auf der anerkannten Methode der Heizlastberechnung gemäß DIN EN 12831-1:2017, Abschnitt 6, dem detaillierten Standardverfahren. Dieses Verfahren stellt eine sehr präzise Grundlage für die Auslegung von Heizungsanlagen dar, setzt jedoch eine umfassende Kenntnis der geometrischen und wärmetechnischen Parameter voraus. Während es in bestimmten Kontexten, wie bei anlagentechnischen Änderungen oder bei der Bewertung im Gebäudebestand, zu einem hohen Aufwand führen kann, werden in der DIN EN 12831-1:2017 auch vereinfachte Verfahren in den Abschnitten 7 und 8 vorgestellt. Diese sind Anpassungen des ausführlichen Standardverfahrens und eignen sich für Situationen, in denen weniger Details verfügbar oder notwendig sind. Es ist wichtig zu betonen, dass diese Spezifikation sowohl das detaillierte als auch die vereinfachten Verfahren abdeckt und darüber hinaus in einem speziellen Abschnitt ein Verfahren zur groben Bestimmung der Gebäudeheizlast durch Messungen von Wärmemengen oder Verbrauch vorsieht.
Bauelemente eines Gebäudes: Wie beeinflussen Sie Wärmeverluste?
Ein Gebäude ist viel mehr als nur vier Wände und ein Dach. Es besteht aus einer Vielzahl von Elementen und Materialien, die alle einen unterschiedlichen Einfluss auf die Heizlast und die Energieeffizienz haben. Der Schlüssel zur Optimierung des Energieverbrauchs und zur Verringerung des Wärmeverlusts liegt im Verständnis der Rolle jedes dieser Bauelemente.
Wärmeverluste durch die Gebäudehülle
Einfach gesagt entsteht der Wärmeverlust aus einer Kombination von:
Transmissionswärmeverlust - Verluste durch die Gebäudehülle
Diese Verluste entstehen durch die Wärmeleitung innerhalb der Bauteile in der Gebäudehülle. Sie werden berechnet, indem die Fläche jedes Bauteils mit dessen Wärmedurchgangskoeffizienten (U-Wert) und dem Temperaturunterschied zwischen innen und außen multipliziert wird.
Lüftungswärmeverlust - Verluste durch Lüftung
Diese Verluste werden durch den Luftaustausch zwischen dem Innenraum des Gebäudes und der Außenluft hervorgerufen. Die Menge der verlorenen Wärme hängt von der Menge der ausgetauschten Luft, dem Temperaturunterschied zwischen Innen- und Außenluft und der spezifischen Wärmekapazität der Luft ab.
Zusatz-Aufheizleistung - Zusätzlich erforderliche Leistung durch unterbrochenen Heizbetrieb, z.B. Nachtabsenkung
Wenn die Heizung während der Nachtstunden oder zu anderen Zeiten heruntergeregelt oder abgeschaltet wird, um Energie zu sparen, kühlt das Gebäude natürlich ab. Nach dieser Heizpause muss, um die gewünschte Raumtemperatur wieder zu erreichen, eine höhere Energiezufuhr erfolgen als im regulären Heizbetrieb. Diese zusätzliche Energiezufuhr wird als Zusatz-Aufheizleistung bezeichnet.
Durch das sogenannte “Hüllflächenverfahren” wird die Gesamtheizlast des Gebäudes berechnet, indem man die Transmissions- und Lüftungswärmeverluste unf ggf. Zusatz-Aufheizleistung zusammenfasst. Das Verfahren bestimmt die Heizlast eines Gebäudes anhand der Wärmeverluste, die durch die Gebäudehülle (d.h. Außenwände, Dach, Fenster, Türen, Böden gegen unbeheizte Räume oder das Erdreich) auftreten. Doch wie setzt sich diese Gebäudehülle zusammen?
Gebäudegeometrie und -größe
Je größer die Grundfläche und das Volumen eines Gebäudes sind, desto mehr Fläche gibt es potenziell, durch die Wärme entweichen kann. Ein großes Gebäude mit vielen Räumen und einer weitläufigen Grundfläche wird in der Regel mehr Energie benötigen, um beheizt zu werden, als ein kleineres Gebäude mit ähnlichen Isolationseigenschaften. Die Form des Gebäudes kann die Anzahl der Wärmebrücken beeinflussen. Eine Wärmebrücke ist ein Bereich in einer Gebäudehülle (Balkonanschlüsse, Fensterrahmen, Lüftungskanäle), in dem die Wärme schneller übertragen wird als in den benachbarten Bereichen. Komplexe Gebäudeformen, wie beispielsweise solche mit vielen Ecken, Vorsprüngen oder Einbuchtungen, können mehr potenzielle Wärmebrücken aufweisen als einfache, kompakte Gebäudeformen.
Beispiel: Stellen Sie sich zwei Gebäude vor. Das erste ist ein einfaches rechteckiges Gebäude ohne viele architektonische Besonderheiten. Das zweite Gebäude hat die gleiche Grundfläche, ist aber viel komplexer gestaltet, mit mehreren Erkern, Balkonen und unterschiedlichen Dachformen.
Beide Gebäude haben die gleiche Grundfläche, aber das zweite Gebäude, aufgrund seiner komplexen Form, hat mehr Außenfläche (z.B. Wände und Dach), durch die Wärme entweichen kann. Zudem bieten die vielen architektonischen Details wie Erker und Balkone potenzielle Stellen für Wärmebrücken. Daher könnte das zweite Gebäude, selbst wenn es die gleiche Isolation wie das erste Gebäude hat, einen höheren Heizbedarf haben.
Wichtige Bestandteile der Gebäudehülle
- Außenwände: Die Außenwände eines Gebäudes sind die primären Barrieren, die den Innenraum von den externen klimatischen Bedingungen trennen. Ihre Konstruktion, Dicke und die verwendeten Materialien haben einen signifikanten Einfluss darauf, wie viel Wärme aus dem Gebäude nach außen oder von außen nach innen übertragen wird. Verschiedene Materialien haben unterschiedliche Wärmeleitfähigkeiten. So leitet beispielsweise Beton Wärme besser als Holz. Das bedeutet, dass eine Wand aus reinem Beton ohne zusätzliche Isolierung im Winter mehr Wärme aus dem Inneren des Gebäudes nach außen leiten würde als eine Holzwand. Die Dicke der Wand spielt ebenfalls eine Rolle. Eine dickere Wand bietet in der Regel einen besseren Wärmewiderstand als eine dünnere. Dämmmaterialien sind speziell darauf ausgelegt, den Wärmefluss zu minimieren. Sie haben eine geringe Wärmeleitfähigkeit und können somit den Transmissionswärmeverlust einer Außenwand erheblich reduzieren. Das bedeutet, dass trotz kälterer Außentemperaturen die Innentemperatur eines gut isolierten Gebäudes stabil gehalten werden kann, ohne dass die Heizsysteme übermäßig beansprucht werden.
- Fenster und Außentüren: Fenster und Außentüren sind oft kritische Bereiche in Bezug auf den Wärmeverlust eines Gebäudes. Während die Wände, das Dach und der Boden oft mit dicken Dämmschichten ausgestattet sind, können Fenster und Türen, wenn sie nicht korrekt gewählt und installiert werden, zu einem erheblichen Energieverlust führen. Einfachverglasungen bestehen nur aus einer einzelnen Glasschicht und bieten daher kaum Widerstand gegen Wärmeübertragung. Das Resultat ist ein hoher Wärmeverlust, was insbesondere in kälteren Monaten zu hohen Heizkosten führen kann. Doppelverglasungen, wie der Name schon sagt, verwenden zwei Glasschichten, die durch einen Raum getrennt sind. Dieser Zwischenraum, oft gefüllt mit einem Inertgas wie Argon, fungiert als Isolierschicht und reduziert den Wärmeverlust erheblich. Dreifachverglasungen gehen noch einen Schritt weiter und verwenden drei Glasschichten. Außentüren spielen eine ebenso wichtige Rolle wie Fenster, wenn es um den Wärmeschutz eines Gebäudes geht. Auch wenn sie in der Regel eine kleinere Fläche im Vergleich zu den Fenstern ausmachen, können schlecht isolierte oder undichte Türen erhebliche Wärmeverluste verursachen. Die meisten modernen Außentüren bestehen aus einem Kern aus isolierendem Material, der von haltbaren Außenmaterialien wie Holz, Metall oder Kunststoff umgeben ist. Die Materialauswahl beeinflusst nicht nur das Aussehen und die Sicherheit der Tür, sondern auch ihre Isoliereigenschaften. Beispielsweise bieten Massivholztüren oft eine gute natürliche Isolation, während Metalltüren, sofern sie nicht mit einem thermischen Unterbrecher ausgestattet sind, zu höheren Wärmeverlusten führen können. Ein oft übersehener Aspekt von Außentüren sind die Dichtungen, welche verschlissen oder undicht sind. Eine gut gewählte und eingebaute Türschwelle kann ebenfalls dazu beitragen, den Wärmeverlust zu minimieren und Zugluft zu verhindern.
- Dach und Decken: Das Dach eines Gebäudes hat die herausfordernde Aufgabe, gegen alle Widrigkeiten des Wetters zu schützen, von der sengenden Sommersonne bis zu kaltem Winterfrost. Gerade weil Wärme naturgemäß nach oben steigt, kann ein unzureichend gedämmtes Dach zu einem der Hauptverlustpunkte für die im Gebäude erzeugte Wärme werden. Ein Dach besteht in der Regel aus mehreren Schichten, angefangen bei der Außenbedeckung (z. B. Ziegel, Schiefer oder Blech) über eine Unterdeckung, Dämmmaterialien bis hin zur Innendecke. Die Dämmung spielt dabei die Schlüsselrolle. Moderne Dämmmaterialien wie Mineralwolle, Zellulose oder Holzfasern haben hervorragende Isoliereigenschaften und können den Wärmeverlust erheblich reduzieren. Die Neigung des Daches kann ebenfalls einen Einfluss auf den Wärmeverlust haben. Ein steil geneigtes Dach hat eine größere Oberfläche als ein flaches Dach und bietet daher potenziell mehr Raum für Wärmeverlust, insbesondere wenn es nicht richtig gedämmt ist. Allerdings kann die Neigung auch Vorteile bieten, z. B. durch eine bessere Abführung von Regenwasser oder Schnee. Die Decken in einem Haus, insbesondere die Decke des obersten Geschosses, die direkt an das Dach grenzt, sind ebenfalls für den Wärmeschutz von Bedeutung. Hier können Wärmebrücken entstehen, etwa durch Durchdringungen für Licht oder Lüftung, die den Wärmeverlust erhöhen. Es spielt also eine Rolle, ob die Decke an einen beheizten oder unbeheizten Raum angrenzt.
- Fußböden: Fußböden sind jene Teile eines Gebäudes, die ständig in direktem Kontakt mit den Bewohnern stehen. Ein kalter Fußboden kann daher direkt unser Wohlgefühl beeinträchtigen und gleichzeitig ein Indikator für ineffiziente Wärmenutzung sein. Fußböden, die direkt auf dem Erdreich liegen oder in Kontakt mit unbeheizten Kellerräumen stehen, sind besonders anfällig für Wärmeverluste. Das Erdreich hat eine konstante, meist niedrige Temperatur und kann daher, wenn keine ausreichende Isolation vorhanden ist, die Wärme aus den darüberliegenden Räumen abführen. In unbeheizten Kellerräumen kann kalte Luft zirkulieren, die ebenfalls Wärme aus den Fußböden der darüberliegenden Räume zieht. Eine effektive Isolationsschicht unter dem Fußboden, z.B. aus Zellulose oder Holfasern, kann die Wärmeübertragung zum Erdreich oder zum Keller hin erheblich reduzieren. Auch die Art des Fußbodenbelags kann zur Wärmedämmung beitragen: Teppiche und Holzböden bieten eine zusätzliche Isolationsschicht, während Fliesen, obwohl sie angenehm kühl im Sommer sind, im Winter mehr Wärme leiten können.
Bedeutung von Bauzustand und Wärmedämmmaßnahmen
Der Zustand, in dem sich ein Gebäude befindet, beeinflusst maßgeblich den Wärmeverlust. Altbauten, die vor der Einführung moderner Bauvorschriften und Techniken errichtet wurden, neigen dazu, weniger energieeffizient zu sein als Neubauten. Daher ist es bei Altbausanierungen besonders wichtig, die Wärmedämmmaßnahmen zu überprüfen und gegebenenfalls zu optimieren.
Der Zustand eines Gebäudes ist entscheidend dafür, wie effizient es seine Wärme hält. Vor allem Altbauten, die oft noch vor strengeren energetischen Standards errichtet wurden, weisen in vielen Fällen deutliche Schwächen in der Wärmedämmung auf. So kann beispielsweise durch undichte Fenster oder schlecht isolierte Wände unnötig Energie verloren gehen. Das Resultat: Höhere Heizkosten und ein unangenehmes Raumklima.
Wenn man in einen Altbau zieht oder ein solches Gebäude besitzt, sind die Wärmedämmmaßnahmen oft der Schlüssel zu deutlichen Energieeinsparungen. Wärmedämmmaßnahmen können in Form von zusätzlicher Isolierung, Austausch von Fenstern oder Türen, oder auch der Anwendung spezieller Dichtungsmethoden erfolgen. Diese Maßnahmen reduzieren den Wärmeverlust und helfen, die Heizlast und damit auch die Heizkosten zu senken. Denken Sie an ein altes Stadthaus, dessen schöne, aber alte Holzfenster vielleicht charmant aussehen, jedoch ständig kalte Luft hereinlassen. Der Austausch solcher Fenster gegen moderne, doppelt oder dreifach verglaste Modelle kann den Wärmedurchgang erheblich reduzieren und somit für ein wärmeres Zuhause bei niedrigeren Heizkosten sorgen.
Wenn keine genaueren Daten zu den Wärmedurchgangskoeffizienten einzelner Bauteile vorliegen, werden die U-Werte gemäß DIN 12831 anhand der der Bautypologie bestimmt.
Heizflächenoptimierung: Wie beeinflusst die Heizflächenauslegung den Energieverbrauch?
Die Heizflächenauslegung spielt eine wichtige Rolle, wenn es darum geht, wie effizient ein Gebäude beheizt wird. Nicht nur die Wahl der richtigen Heizkörper oder Wärmeübertrager, sondern auch ihre korrekte Dimensionierung hat einen direkten Einfluss auf den Energieverbrauch und die entstehenden Heizkosten.
Wahl des Heizsystems
Es gibt verschiedene Arten von Heizsystemen, von herkömmlichen Radiatorheizkörpern bis hin zu Fußboden- oder Wandheizungen. Jedes System hat seine eigenen Vor- und Nachteile in Bezug auf die Wärmeübertragung.
- Heizkörper: Klassische Heizkörper erzeugen Konvektionswärme, bei der warme Luft aufsteigt und kalte Luft absinkt. Dies kann zu Temperaturunterschieden im Raum führen, insbesondere zwischen Boden und Decke. Heizkörper sind gerade in vielen älteren Gebäuden die gängige Form der Wärmeabgabe. Sie sind in der Regel schnell aufgeheizt und können in kürzester Zeit Wärme an den Raum abgeben. Ihr Wirkungsgrad und ihre Effizienz hängen jedoch stark von ihrer Größe, ihrem Design und ihrer Platzierung im Raum ab. Oft werden sie unter Fenstern platziert, um Kaltluftströme auszugleichen, was jedoch zu Wärmeverlusten führen kann.
- Fußboden- und Wandheizungen: Diese Systeme verteilen die Wärme über eine größere Fläche und nutzen dabei Strahlungswärme. Dadurch entsteht eine gleichmäßigere Temperaturverteilung im Raum, was den Komfort erhöht und den Energieverbrauch reduziert, da weniger Temperaturschwankungen ausgeglichen werden müssen. Korrekt dimensioniert, kann eine Fußbodenheizung zu einem geringeren Energieverbrauch führen, da sie bei niedrigeren Vorlauftemperaturen effizient arbeitet. Ein zusätzlicher Vorteil ist, dass sie unsichtbar ist und somit keinen anderweitig nutzbaren Raum beansprucht.
Passende Dimensionierung
Eine Über- oder Unterdimensionierung der Heizflächen kann den Energieverbrauch erheblich beeinflussen. Ein zu großer Heizkörper in einem kleinen Raum wird die Temperatur schnell ansteigen lassen, was zu einem übermäßigen Energieverbrauch führen kann. Umgekehrt kann ein zu kleiner Heizkörper in einem großen Raum nicht genügend Wärme liefern, was dazu führt, dass das Heizsystem ständig läuft, um die gewünschte Temperatur zu erreichen.
Die Dimensionierung der Heizflächen beeinflusst daher maßgeblich die Effizienz und den Energieverbrauch eines Heizungssystems. Gerade, wenn man von fossilen Brennstoffen unabhängig werden und verstärkt auf Wärmepumpen als Heizquelle zurückzugreifen möchte, wird die korrekte Auslegung der Heizflächen umso relevanter.
Alte Gebäude sind häufig mit Heizsystemen ausgestattet, die auf einer hohen Vorlauf- und Rücklauftemperatur basieren, beispielsweise 75°C und 65°C. Wärmepumpen hingegen arbeiten besonders effizient bei niedrigen Vorlauftemperaturen. Daher ist es für ihre effiziente Nutzung wichtig, dass die Wärmeabgabe im Gebäude über Heizflächen erfolgt, die auch bei niedrigeren Temperaturen eine angemessene Wärmeleistung liefern können.
Die Begriffe "Vorlauf" und "Rücklauf" beziehen sich auf den Kreislauf des Heizungswassers in einem Heizungssystem.
- Vorlauftemperatur: Diese bezeichnet die Temperatur des Wassers, wenn es aus dem Wärmeerzeuger (z.B. einem Heizkessel oder einer Wärmepumpe) kommt und in das Heizsystem, wie Heizkörper oder Fußbodenheizung, eintritt. Das heißt, das Wasser hat die Vorlauftemperatur, wenn es auf dem Weg ist, die Wärme in den Räumen abzugeben. Eine höhere Vorlauftemperatur bedeutet meistens auch einen höheren Energieverbrauch, da das Wasser stärker erhitzt werden muss.
- Rücklauftemperatur: Nachdem das Heizungswasser seine Wärme an die Räume abgegeben hat, fließt es zurück zum Wärmeerzeuger, um erneut erhitzt zu werden. Die Temperatur, die das Wasser zu diesem Zeitpunkt hat, wird als Rücklauftemperatur bezeichnet. Sie ist in der Regel niedriger als die Vorlauftemperatur, da das Wasser beim Durchfließen der Heizkörper oder der Fußbodenheizung abkühlt.
Die Differenz zwischen Vorlauf- und Rücklauftemperatur wird auch als "Spreizung" bezeichnet. Eine optimierte Spreizung ist ein Indikator für ein gut eingestelltes Heizsystem. Das Verhältnis von Vorlauf- und Rücklauftemperatur ist ausschlaggebend.
Eine Fußbodenheizung kann aufgrund ihrer großflächigen Ausbreitung bereits bei geringen Temperaturen für eine angenehme Raumtemperatur sorgen kann. In Gebäuden mit klassischen Heizkörpern hingegen sollte genau geprüft werden, ob die vorhandenen Heizflächen auch bei niedriger Vorlauftemperatur die benötigte Wärme bereitstellen können.
Die Dimensionierung der Heizflächen hat direkte Auswirkungen auf die Vorlauftemperatur, die ein Heizsystem benötigt. Das Herzstück einer Wärmepumpe, der Verdichter, erhöht die Temperatur des Kältemittels. Je geringer die Temperaturdifferenz zwischen der Wärmequelle (z.B. Erdreich oder Außenluft) und der benötigten Vorlauftemperatur ist, desto effizienter kann die Wärmepumpe arbeiten.
Wenn zum Beispiel in einem Gebäude mit klassischen Heizkörpern die Vorlauftemperatur verringert wird, könnten diese Heizkörper in manchen Räumen nicht ausreichend Wärme abgeben. Daher ist es wichtig, im Vorfeld genau zu ermitteln, welche Heizleistung in den einzelnen Räumen benötigt wird und ob die bestehenden Heizkörper diese auch bei geringerer Vorlauftemperatur noch liefern können. In einigen Fällen könnte es notwendig sein, größere Heizkörper zu installieren oder das Heizsystem grundlegend zu überdenken.
Raumweise Heizlastberechnung: Wie ermittelt man die optimale Wärmeversorgung?
Die Raumweise Heizlastberechnung ist ein wesentlicher Schritt zur Ermittlung des Wärmebedarfs eines Gebäudes und der optimalen Wärmeversorgung. Sie ermöglicht es, den Wärmebedarf jedes einzelnen Raumes genau zu bestimmen und trägt damit zu einer effizienten und kosteneffektiven Heizungsplanung bei.
In jedem Gebäude sind Wärmeverluste unvermeidlich. Sie entstehen durch die weiter oben beschriebenen Faktoren, die direkt mit den baulichen Eigenschaften und der Nutzung des Gebäudes zusammenhängen. Der Transmissionswärmeverlust ist einer dieser Faktoren, der direkt durch die Gebäudehülle, wie Außenwände, Dach und Fenster, auftritt. Um diesen Verlust zu berechnen, ist das Verständnis von U-Wert und Wärmedurchgangskoeffizienten entscheidend. Diese Werte bieten Einblicke in die Isolationseffizienz der Bauteile, und damit, wie gut sie Wärme bewahren oder verlieren können.
Parallel dazu tritt der Lüftungswärmeverlust auf, welcher den Wärmeverlust durch den Luftaustausch zwischen dem Innenraum des Gebäudes und der Außenluft beschreibt. Dieser Verlust wird maßgeblich durch den Luftwechsel beeinflusst, welcher wiederum von der Gebäudedichtheit und dem Raumvolumen abhängt.
Schließlich gibt es noch die Zusatz-Aufheizleistung, die sich insbesondere auf die Speichermasse des Gebäudes bezieht. Die Speichermasse eines Gebäudes - ob leicht oder schwer - spielt eine entscheidende Rolle, wenn es darum geht, wie schnell ein Gebäude nach einer Absenkung wieder aufgeheizt werden muss.
Es ist somit offensichtlich, dass das richtige Verständnis dieser drei Komponenten - Transmissionswärmeverlust durch U-Werte, Lüftungswärmeverlust durch Luftwechsel und Zusatz-Aufheizleistung durch die Speichermasse - unerlässlich ist, um die optimale Wärmeversorgung eines Gebäudes zu bestimmen. Im Folgenden gehen wir näher auf diese Faktoren ein und beleuchten insbesondere, wie sie in der Raumweise Heizlastberechnung berücksichtigt werden.
Methoden zur präzisen Heizlastberechnung
Es gibt verschiedene Methoden, um die Heizlast eines Raumes oder eines gesamten Gebäudes zu berechnen. Die Grundidee ist dabei immer dieselbe: Es wird ermittelt, wie viel Wärme benötigt wird, um eine bestimmte Innentemperatur aufrechtzuerhalten, während draußen kältere Temperaturen herrschen. Um diesen Wärmebedarf zu ermitteln, werden Aspekte wie die Dämmqualität des Gebäudes, die Art und Qualität der Fenster und Türen, sowie die Größe und Ausrichtung der Räume berücksichtigt. Spezialisierte Softwareprogramme, wie autarc, helfen dabei, diese komplexen Berechnungen durchzuführen. Mehr hierzu in unserem Blogbeitrag: Die besten Softwarelösungen für die Wärmepumpen-Planung im Vergleich.
Bedeutung von U-Wert und Wärmedurchgangskoeffizienten: Kernfaktoren der Heizlastberechnung
In der Heizlastberechnung spielen Begriffe wie der U-Wert und der Wärmedurchgangskoeffizient eine entscheidende Rolle. Der U-Wert gibt an, wie gut ein Bauteil isoliert ist. Je niedriger der U-Wert, desto besser die Dämmung. Der Wärmedurchgangskoeffizient hingegen beschreibt, wie viel Wärme durch ein Bauteil übertragen wird. Diese beiden Werte sind unerlässlich, um zu verstehen, wie viel Wärme ein Gebäude verliert und wie viel zugeführt werden muss, um eine gewünschte Innentemperatur zu erreichen.
U-Werte: Diese zeigen, wie viel Wärme bei einer Temperaturdifferenz von 1°C über 1m² Fläche eines Bauteils (wie Fenster, Tür, Decke) übertragen wird. Ein niedriger U-Wert steht dabei für eine bessere Isolierung des Bauteils. Formel: U = 1 / (Summe der Widerstände der Schichten)
Auch wichtig:
- Unbekannte U-Werte: Wenn die genauen U-Werte unbekannt sind, werden typische Werte basierend auf dem Alter des Bauteils aus Tabellen der DIN EN 12831 herangezogen (verschiedene Baualtersklassen von 1918 bis 1995).
- Pauschaler Wärmebrückenzuschlag: Es wird ein standardmäßiger Zuschlag von 0,10 W/m²·K für Wärmebrücken berücksichtigt, um unerwartete Wärmeverluste zu kompensieren.
- Raumtemperatur: Eine einheitliche Raumtemperatur von 20°C wird angenommen, sofern keine anderen spezifischen Werte vereinbart werden.
- Vereinfachte Temperaturkorrekturfaktoren: Abhängig von der Lage des Bauteils, z.B. ob es an einen unbeheizten Raum oder das Erdreich grenzt, werden standardisierte Korrekturfaktoren verwendet.
Luftwechsel: Die Bestimmung des Lüftungswärmeverlusts erfordert Kenntnisse darüber, wie intensiv und häufig ein Raum gelüftet wird. Hierbei werden Faktoren wie die Gebäudedichtheit und das Raumvolumen berücksichtigt. Für Industriehallen oder Gebäudeanlagen mit Lüftungsanlagen müssen zusätzliche Überlegungen angestellt werden.
Die Luftwechselrate gibt an, wie oft pro Stunde das gesamte Raumvolumen durch neue Luft ersetzt wird. Es ist jedoch wichtig zu beachten, dass diese Rate nicht unbedingt bedeutet, dass der gesamte Raum mit Frischluft durchflutet wird. Dies liegt daran, dass die Raumgeometrie ebenfalls einen erheblichen Einfluss auf die Verteilung der zugeführten Außenluft hat.
In Situationen, in denen die anwesenden Personen die Hauptquelle für luftfremde Emissionen sind, wie z.B. in Büros oder Lagerhallen, kann eine Luftwechselrate von 1/h durch freie Lüftung sowohl erreichbar als auch ausreichend sein. Das bedeutet, dass die gesamte Raumluft in einer Stunde einmal erneuert wird.
Auch wichtig:
- Dichtheit des Gebäudes: Basierend auf der angenommenen Gebäudedichtheit werden die Richtwerte angenommen, wie “bestehendes Gebäude - dicht” [n = 0,25 h/1], “bestehendes Gebäude - weniger dicht” [n = 0,5 h/1], und “bestehendes Gebäude - undicht” [n = 1,0 h/1].
- Volumenberechnung: Das Volumen des Gebäudes muss ermittelt werden, um den Lüftungswärmeverlust präzise zu berechnen. Hierbei wird die Norm-Außentemperatur gemäß DIN EN 12831 verwendet.
Speichermasse: Speichermasse bezieht sich auf die Fähigkeit eines Baustoffs oder einer Baustruktur, Wärme zu speichern und langsam wieder abzugeben. Materialien mit hoher Dichte, wie Stein, Beton oder Ziegel, haben in der Regel eine hohe Speichermasse. Wenn sie einmal aufgeheizt sind, geben sie diese Wärme langsam über Stunden oder sogar Tage hinweg wieder ab, selbst wenn die Heizquelle nicht mehr aktiv ist. Das Vorhandensein von massiven Bauteilen mit hoher Speichermasse im Gebäude kann dazu führen, dass die benötigte Aufheizleistung reduziert wird. Das liegt daran, dass diese Materialien dazu neigen, die Wärme über einen längeren Zeitraum zu speichern und gleichmäßig abzugeben, wodurch Temperaturschwankungen verringert werden. Umgekehrt bedeutet dies, dass Gebäude mit geringerer Speichermasse schneller auf Temperaturänderungen reagieren und daher möglicherweise eine höhere Aufheizleistung benötigen, um eine konstante Innentemperatur aufrechtzuerhalten.
Auch wichtig:
- Gebäudemasse: Eine leichte Gebäudemasse (l) liegt vor bei Konstruktionen mit abgehängten Decken, aufgeständerte Böden oder Wände in Leichtbauweise beispielsweise. Sie speichern weniger Wärme und können daher schneller auf Temperaturänderungen reagieren. Eine mittelschwere oder schwere Gebäudemasse (s) ist bei Bauweisen mit Betondecken, -wänden oder -böden und Mauerwerk der Fall. Sie haben eine höhere Speicherkapazität und geben Wärme über längere Zeiträume ab.
- Wiederaufheizfaktor: Dieser Faktor berücksichtigt, wie schnell ein Raum nach einer Absenkphase wieder auf die gewünschte Temperatur gebracht werden muss. Er ist abhängig von der zuvor genannten Gebäudemasse, dem Luftwechsel und der Dauer und Tiefe der Temperaturabsenkung.
Einbeziehung von Innentemperatur, Auslegungsaußentemperatur und Energiebedarf
Die Differenz zwischen der Innen- und Außentemperatur eines Gebäudes ist ein zentraler Faktor, wenn es darum geht, den Heizbedarf korrekt zu berechnen. Dieser Temperaturunterschied, auch Temperaturgradient genannt, beeinflusst direkt, wie viel Wärme durch die Bauelemente eines Gebäudes fließt. Je größer der Unterschied zwischen Innen- und Außentemperatur, desto größer ist der Antrieb für Wärme, von einem Bereich mit höherer Temperatur zu einem Bereich mit niedrigerer Temperatur zu fließen.
Um die optimale Wärmeversorgung zu bestimmen, muss man die gewünschte Innentemperatur, die Auslegungsaußentemperatur (d.h. die tiefste erwartete Außentemperatur) und den gesamten Energiebedarf des Gebäudes berücksichtigen. Während die Innentemperatur oft auf einen Komfortwert wie 20 Grad Celsius festgelegt wird, kann die Auslegungsaußentemperatur je nach geografischer Lage und lokalem Klima stark variieren. Der Energiebedarf ergibt sich aus der Summe des Wärmebedarfs aller Räume, unter Berücksichtigung aller bautechnischen Eigenschaften und Umgebungsbedingungen.
Beispiel: Angenommen, ein Gebäude befindet sich in einer Region, in der die Auslegungsaußentemperatur gemäß Norm -10°C beträgt. Wenn die gewünschte Innentemperatur des Gebäudes 20°C beträgt, dann beträgt der Temperaturunterschied 30°C.
Für jedes Bauelement (z.B. Außenwand, Fenster) wird der Wärmedurchgangskoeffizient (U-Wert) herangezogen, um den Wärmefluss durch dieses Element zu berechnen. Multipliziert man den U-Wert eines Bauelements mit seiner Fläche und dem Temperaturunterschied, erhält man den Wärmeverlust dieses Elements.
In der Praxis bedeutet dies: Wenn der U-Wert einer Außenwand 0,3 W/(m²·K) ist und die Wand eine Fläche von 100 m² hat, dann beträgt der Wärmeverlust dieser Wand bei einem Temperaturunterschied von 30°C:
0,3 W/(m²·K) × 100 m² × 30 K = 900 W oder 0,9 kW.
Je höher der Temperaturunterschied und je schlechter der U-Wert eines Bauelements, desto höher wird der Wärmeverlust sein.
Von der Heizlast zur Wärmepumpe: Welche Rolle spielt die Dimensionierung bei der Auswahl?
Die Dimensionierung einer Wärmepumpe ist der letzte entscheidende Schritt in der Planung, um ein effizientes und leistungsstarkes Heizsystem in einem Gebäude zu gewährleisten. Was gibt es hierbei zu beachten?
Ein zentrales Problem, das oft übersehen wird, ist die Überdimensionierung von Heizsystemen. Stellen Sie sich eine Wärmepumpe vor, die für ein viel größeres Gebäude konzipiert wurde als das, in dem sie tatsächlich installiert ist. Diese Pumpe würde nicht nur zu viel Energie verbrauchen, sondern auch unnötig hohe Betriebskosten verursachen. Zudem würde sie häufiger ein- und ausschalten, was zu einem erhöhten Verschleiß und somit zu einer verkürzten Lebensdauer führt. Ein zu groß dimensioniertes System kann zudem unerwünschte Temperaturschwankungen im Gebäude verursachen.
Auf der anderen Seite steht die Wärmepumpenleistung, die im Wesentlichen den Energiebedarf eines Hauses an den kältesten Tagen des Jahres decken muss. Wenn die Heizleistung der Wärmepumpe zu gering ist, kann das Haus nicht auf die gewünschte Temperatur gebracht werden, was zu einem unangenehmen Wohnklima führt. Es ist daher notwendig, die richtige Balance zu finden und die Wärmepumpe exakt auf den tatsächlichen Wärmebedarf des Hauses abzustimmen.
Faktoren für die Dimensionierung
Fläche des Gebäudes: Der Wärmebedarf steigt proportional zur Größe des Gebäudes. Hierbei geht es nicht nur um die Grundfläche, sondern auch um die Raumhöhe, da ein höherer Raum mehr Volumen hat, das beheizt werden muss.
Vorverbräuche der Heizung: Das sind die Energiereserven, die bereits im System gespeichert sind, bevor die Wärmepumpe beginnt zu arbeiten. Dies kann aufgrund früherer Heizzyklen oder anderer Energiequellen im System sein.
Dämmzustand des Gebäudes: Ein Gebäude mit effizienter Dämmung behält Wärme besser und vermindert den Wärmebedarf. Detaillierte Untersuchungen des U-Wertes der Bauelemente können hier Aufschluss geben. Der U-Wert gibt an, wie gut die Isoliereigenschaften eines Materials sind.
Regionales Klima: Hier geht es nicht nur um die durchschnittliche Wintertemperatur. Extreme Kälteperioden, Windgeschwindigkeiten und Luftfeuchtigkeit können ebenfalls den Heizbedarf beeinflussen.
Art der Heizelemente: Unterschiedliche Heizelemente haben unterschiedliche Reaktionszeiten und Wärmeübertragungseffizienzen. Eine Fußbodenheizung etwa gibt ihre Wärme überwiegend durch Strahlung ab und reagiert langsamer als ein Heizkörper.
Heizgewohnheiten der Bewohner: Es ist auch wichtig zu wissen, zu welchen Zeiten geheizt wird, ob kontinuierlich oder in Intervallen, und wie hoch die gewünschte Innentemperatur ist.
Die passende Wärmepumpe wählen
Die Auswahl der richtigen Wärmepumpe nach der ermittelten Heizlast folgt einem systematischen Ansatz, der die technischen Anforderungen und die individuellen Bedürfnisse berücksichtigt. Dabei kommt es nicht nur darauf an, ein leistungsstarkes Gerät zu wählen, sondern eines, das genau auf den Bedarf Ihres Hauses abgestimmt ist.
- Ermitteln Sie die Heizlast: Bevor Sie die passende Wärmepumpe auswählen, müssen Sie den Wärmebedarf Ihres Gebäudes kennen. Die genormte Heizlastberechnung nach DIN EN 12831 ist hierfür das gängige Verfahren, das eine präzise und detaillierte Analyse des Wärmebedarfs ermöglicht. Weitere Informationen oben im Artikel.
- Berücksichtigen Sie die Wohnfläche: Multiplizieren Sie den ermittelten Wärmebedarf pro m² Wohnfläche mit der Gesamtfläche Ihres Gebäudes. Dies gibt Ihnen den Gesamtwärmebedarf in kW. Beispielrechnung für einen Altbau: Angenommen, Sie bewohnen einen Altbau ohne Wärmedämmung mit einer Wohnfläche von 120 m², dann wäre der Wärmebedarf:130 m² x 0,12 kW/m² = 15,6 kW. Das bedeutet, für Ihren Altbau benötigen Sie eine Wärmepumpe, die eine Leistung von ungefähr 15,6 kW erbringen kann. Bei der Auswahl sollten Sie auch eventuelle zukünftige Änderungen, wie etwa eine verbesserte Wärmedämmung oder die Erweiterung der Wohnfläche, berücksichtigen. In der Regel werden für gut gedämmte Einfamilienhäuser Wärmepumpen mit einer Größe von 3 bis 12 kW bevorzugt. In einem älteren Altbau oder größeren Gebäuden können jedoch Wärmepumpen mit Leistungen von bis zu 15 oder 16 kW erforderlich sein.
- Verwenden Sie Richtwerte: Wenn Sie noch kein detailliertes Verfahren angewendet haben oder einen schnellen Überblick erhalten möchten, können Sie sich an den Richtwerten der DIN EN 12831 orientieren. Diese Werte sind nützlich, um eine erste Abschätzung des Wärmebedarfs zu erhalten, sollten jedoch zwingend für endgültige Entscheidungen durch eine detaillierte Analyse ergänzt werden (siehe Tabelle unten).
- Überdimensionierung vermeiden: Es ist wichtig, nicht einfach die Wärmepumpe mit der höchsten verfügbaren Leistung auszuwählen. Eine überdimensionierte Wärmepumpe führt zu unnötigen Kosten und kann ineffizient arbeiten, da sie häufiger ein- und ausschaltet. Dies kann auch zu einer verkürzten Lebensdauer der Pumpe führen.
- Zukünftige Änderungen berücksichtigen: Wenn Sie planen, Ihr Gebäude in naher Zukunft zu erweitern oder zusätzliche Isolierung hinzuzufügen, sollten Sie dies bei der Auswahl der Wärmepumpe berücksichtigen. Es kann sinnvoll sein, eine Wärmepumpe mit einer leicht höheren Leistung zu wählen, um zukünftige Anforderungen abzudecken.
- Hersteller und Modelle vergleichen: Es gibt eine Vielzahl von Wärmepumpenherstellern und Modellen auf dem Markt. Vergleichen Sie die technischen Daten, die Energieeffizienz, den Preis und die Kundenbewertungen, um die beste Wahl für Ihr Gebäude zu treffen.
- Einbindung von Fachpartnern: Schließlich ist es immer ratsam, einen Fachmann zu Rate zu ziehen, wenn es um die Auswahl und Installation einer Wärmepumpe geht. Ein Experte kann Sie detailliert beraten und sicherstellen, dass Sie die richtige Wahl treffen.
- Softwareunterstützung: Software ermöglicht Fachpartnern, SHK-Betrieben und Energieberatern durch geführte Abläufe eine sichere und effiziente Arbeitsweise. Insbesondere bei der normgerechten Erfassung von Heizlast, Heizflächen und hydraulischem Abgleich nach Verfahren B bieten sie eine unerlässliche Unterstützung. Alles Prozesse, die früher viel Zeit und Mühe in Anspruch nahmen, werden heute durch Softwarelösungen erheblich beschleunigt. In wenigen Minuten können alle Daten normgerecht erhoben werden und eine erste Vorauswahl der geeigneten Wärmepumpe getroffen werden.
.png)
Fazit
Die Heizlastberechnung ist ein unverzichtbares Instrument auf dem Weg zur Auswahl der idealen Wärmepumpe für Ihr Gebäude. Sie ermöglicht es, den genauen Wärmebedarf zu ermitteln, wodurch Über- oder Unterdimensionierungen vermieden werden.
Die Grundlagen der Heizlastberechnung zeigen auf, warum diese Berechnung für die Effizienz und Funktionalität der Heizung unerlässlich ist. Nur wenn man den exakten Wärmebedarf kennt, kann man auch das passende Heizsystem auswählen.
Diverse Bauelemente eines Gebäudes, wie Fenster, Dächer oder Wände, spielen eine entscheidende Rolle bei Wärmeverlusten. Ihre Beschaffenheit und Qualität können die benötigte Heizleistung erheblich beeinflussen. Ein gut gedämmtes Gebäude etwa benötigt weitaus weniger Heizenergie als ein schlecht isoliertes.
Die Heizflächenoptimierung verdeutlicht, dass nicht nur die Gesamtfläche, sondern auch die Auslegung und Verteilung der Heizflächen im Gebäude den Energieverbrauch prägen. Eine sorgfältige Planung und Anordnung kann zu erheblichen Energieeinsparungen führen.
Und schließlich stellt die Dimensionierung in "Von der Heizlast zur Wärmepumpe" sicher, dass die ausgewählte Wärmepumpe genau dem Bedarf entspricht, den das Gebäude hat. Die genaue Abstimmung von Heizlast und Wärmepumpenleistung ist zentral für eine energieeffiziente und komfortable Raumtemperierung.
Zusammengefasst ist die raumweise Heizlastberechnung der Schlüssel, um sowohl Energieeinsparungen als auch optimalen Wohnkomfort zu gewährleisten. Wer in eine Wärmepumpe investiert, sollte diesen Schritt daher keinesfalls überspringen und im Zweifelsfall auf die Expertise von Fachleuten setzen.
Finden Sie die richtige Wärmepumpe mit autarc!
Suchen Sie nach einer verlässlichen Methode, um die perfekte Wärmepumpe für Ihr Gebäude zu ermitteln? Dann setzen Sie auf die Präzision der raumweisen Heizlastberechnung mit autarc! Unsere fortschrittliche Software macht die komplexe Berechnung und Auswahl leicht verständlich und führt Sie zielsicher zur optimalen Wärmepumpenlösung. Egal, ob Sie vom Fach sind oder sich das erste Mal mit dem Thema befassen, autarc stellt Ihnen alle Werkzeuge zur Verfügung, die Sie benötigen.
Ermitteln Sie die exakte Heizlast Ihres Gebäudes, berücksichtigen Sie alle relevanten Bauelemente und dimensionieren Sie Ihre Wärmepumpe präzise! Zudem können Sie mit autarc herausfinden, welche Fördermittel für Ihr Projekt verfügbar sind und diese direkt über die Software beantragen. Beginnen Sie Ihre Reise zu einem effizienter beheizten Heim und entdecken Sie die Vorteile von autarc.
Klicken Sie hier, um mehr über autarc zu erfahren und direkt loszulegen.